Integration of cellular metabolism is controlled by insulin and the opposing actions of glucagon and epinephrine. When food is available in abundance, or when the body needs to make stored energy available, changes in the circulating levels of these hormones allow the body to respond accordingly. This discussion of metabolic regulation will be tailored to fit two scenarios, the fed and fasted states, and highlight how metabolite flux is modulated by the direct activating or inhibiting of enzymes, or by inducing or repressing their transcription in the nucleus.
- Introduction
Five major organs play dominant roles in fuel metabolism:
- Liver
- Adipose
- Muscle
- Pancreas
- Brain
Each of these tissues possesses a unique repertoire of enzymes allowing them to differentially store, use, or generate specific fuels that the body needs. These tissues form a network with each other and do not function in isolation, and it is through this communication that one tissue is able to provide substrates to another. The nervous system mediates communication between tissues, along with circulating substrate availability and levels of plasma hormones.
Hormones induce the up- or down-regulation of the transcription of key enzymes in all pathways of intermediary metabolism.
Catabolism
Anabolism
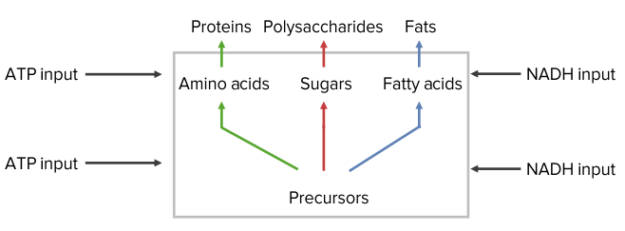
Hormones
Insulin
Insulin is a polypeptide hormone produced by β-cells in the pancreatic islets of Langerhans. Its biosynthesis involves the production of two inactive precursors preproinsulin and proinsulin, which will be subsequently cleaved to form the active hormone upon a rise in blood glucose levels. Insulin causes glucose uptake in both myocytes and adipocytes and directs these cells to synthesize glycogen, protein, and triacylglycerols. These actions are mediated by insulin’s binding to the α-subunit of the insulin receptor, which produces a cascade of cell-signaling β-subunit of insulin receptor substrate (IRS) proteins.
Effect of insulin on glucose uptake and metabolism. Insulin binds to its receptor (1), which in turn starts many protein activation cascades (2). These include translocation of Glut-4 transporter to the plasma membrane and influx of glucose (3), glycogen synthesis (4), glycolysis (5) and fatty acid synthesis (6). It works at other sizes, but sometimes truncates the text on the far right.
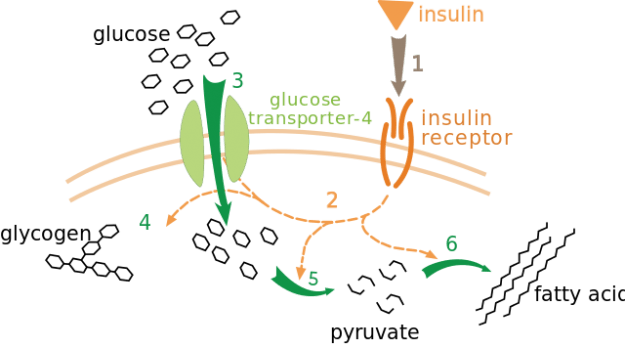
Effect of insulin on glucose uptake and metabolism. Insulin binds to its receptor (1), which in turn starts many protein activation cascades (2). These include translocation of Glut-4 transporter to the plasma membrane and influx of glucose (3), glycogen synthesis (4), glycolysis (5) and fatty acid synthesis (6). It works at other sizes, but sometimes truncates the text on the far right.
Glucagon
Glucagon is a polypeptide hormone as well, produced by the α-cells of the pancreatic islets, in response to low blood glucose. Along with epinephrine (produced in the adrenal cortex), cortisol, and growth hormone, it acts to oppose insulin release, secretion, and uptake by peripheral tissues. These counter-regulatory hormones maintain blood glucose levels during the period of hypoglycemia, extreme exercise, or survival situations in which the “fight-or-flight” response is invoked.
Metabolic network showing the links between enzymes and metabolites that interact with the Arabidopsis TCA cycle KEGG classification M00009. Enzymes and metabolites are the nodes (red), interactions are the lines. In total, 43 enzymes and 40 metabolites are shown.
Glucagon increases the amount of available glucose by inducing glycogenolysis, gluconeogenesis, lipolysis, ketone body synthesis, and the uptake of amino acids by the liver. Free fatty acids released through lipolysis undergo β-oxidation, forming acetyl-CoA, which can enter the TCA cycle or be converted into ketone bodies.
Glucagon binds to high-affinity G-protein-coupled receptors that reside on the membranes of hepatocytes, resulting in the activation of the cyclic AMP (cAMP)-producing enzyme adenylyl cyclase. This second messenger acts to activate cAMP-dependent protein kinase A (PKA), which results in the phosphorylation-mediated activation or inhibition of the regulatory enzymes involved in carbohydrate and lipid metabolism. Note that the receptors that bind glucagon are different than the types that bind epinephrine or insulin and are not found in skeletal muscle.
Also employed to combat hypoglycemia is the hypothalamus, which has glucoreceptors to trigger the autonomic nervous system to secrete epinephrine, and the anterior pituitary to release adrenocorticotropic hormone (ACTH) and growth hormone (GH). ACTH increases cortisol synthesis and releases in the adrenal cortex. Catecholamines such as epinephrine and norepinephrine playing a supporting role in the fasted state as well, by causing similar cellular responses as those produced by glucagon.
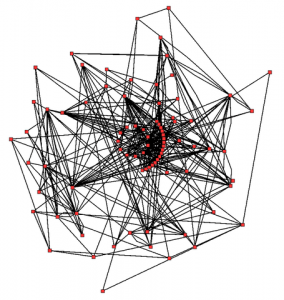
Metabolic network showing the links between enzymes and metabolites that interact with the Arabidopsis TCA cycle KEGG classification M00009. Enzymes and metabolites are the nodes (red), interactions are the lines. In total, 43 enzymes and 40 metabolites are shown.
Mechanisms of Metabolic Regulation
Four mechanisms dictate the flux of metabolites through their respective pathways:
- Substrate availability
- Allosteric enzyme regulation
- Covalent enzyme modification
- Regulation of enzyme synthesis, namely through the induction or repression of transcription.
Allosteric enzyme regulation occurs at rate-determining steps primarily. For example, following meal glycolysis in the liver is stimulated by the increase of fructose-2, 6-bisphosphate, which is an allosteric activator of phosphofructokinase-1 (PFK-1). In addition, the opposing pathway of gluconeogenesis is turned off by fructose-2, 6-bisphophate allosterically inhibiting fructose-1,6-bisphosphatase.
The addition or removal of phosphate groups from specific enzyme serine, threonine, or tyrosine residues allow for enzyme regulation through covalent modification. During the fed state, most of the enzymes regulated in this way will be dephosphorylated and active. Three notable exceptions to this generality are glycogen phosphorylase kinase, glycogen phosphorylase, and hormone-sensitive lipase, which are inactive when they are dephosphorylated. Note that these three enzymes play key roles in releasing stored energy, and so it makes sense to have them inactive during times when metabolites are in abundance.
Image: “A diagram showing at which stages in the DNA-mRNA-protein pathway expression can be controlled.” by ArneLH. License: CC BY-SA 3.0
Regulating enzyme synthesis by inducing or suppressing transcription allows the cell to increase or decrease the total number of key proteins of metabolic pathways, in contrast to allosteric or covalent modifications, which influence the efficiency of enzymes. This process is mediated by proteins that are typically activated or inhibited in the cytosol, usually by phosphorylation or dephosphorylation, and enter the nucleus to induce transcription of regulatory enzymes.
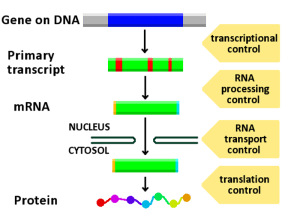
Image: “A diagram showing at which stages in the DNA-mRNA-protein pathway expression can be controlled.” by ArneLH. License: CC BY-SA 3.0
Example of Metabolic Regulation
For example, under fed conditions insulin induces both glycolysis and the pentose phosphate pathway. Protein phosphatase 2A (PP2A) is activated by xylulose-5-phosphate to dephosphorylate Carbohydrate response element-binding protein (ChREBP), which allows it to translocate into the nucleus and bind to Max-like protein (MLX) and induces the transcription of glycolytic enzymes (Hexokinase, PFK-1, PFK-2, Pyruvate kinase) through interaction with carbohydrate response element (ChORE). ChREBP also influences lipogenesis by enhancing the synthesis of Fatty acid synthase (FAS) and Stearoyl-CoA desaturase (SCD).
Image: “Stage 2 of the oxidative pentose phosphate pathway (OPPP): conversion of ribulose-5-phosphate into intermediates of glycolysis.” by C. Muessig. License: CC BY-SA 3.0
In addition, PP2A-mediated dephosphorylation of Sterol regulatory element-binding protein (SREBP1c) in response to insulin drives the cell to generate triacylglycerides from acetyl-CoA, by up-regulating the transcription of Acetyl-CoA carboxylase (ACC), FAS and SCD). SREBP1c is the master regulator of hepatic lipid metabolism.
Insulin also activates protein kinase B (PKB) to phosphorylate another protein, Forkhead box protein 01 (FOXO1). The result of this is that nuclear translocation is blocked, and no gluconeogenic enzymes (G6Pase and PEPCK) are produced because phosphorylated FOXO1 is sent through the ubiquitin pathway to be destroyed.
In the fasted state, glucagon is released by pancreatic α-cells, which activates both PP2A and adenylyl cyclase. PP2A dephosphorylates (FOXO1), which promotes its translocation to the nucleus to induce the transcription of gluconeogenic enzymes G6Pase and PEPCK. Adenylyl cyclase produces cAMP, which induces the phosphorylation and subsequent inactivation ChREBP by Protein kinase A (PKA). In this scenario, the enzymes of glycolysis and lipogenic pathways are attenuated, and the synthesis of enzymes involved in gluconeogenesis is up-regulated.
A sketch of activation effects of cAMP on Protein Kinase A
Covalent modifications occurring during the fasted state include the phosphorylation and activation of glycogen phosphorylase kinase, glycogen phosphorylase, and hormone-sensitive lipase, which allow the cell to mobilize stored glucose as well as lipids for breakdown and energy production when food is scarce or when the body needs to expend a lot of energy for a sustained period.
Other enzymes, such as pyruvate dehydrogenase (PDH), are inactive when phosphorylated. The resulting inhibition of PDH prevents muscle and other tissues from catabolizing glucose and gluconeogenesis precursors. Metabolism shifts toward fat utilization, while muscle protein breakdown to supply gluconeogenesis precursors is minimized, and available glucose is spared for use by the brain.
In summary, the body employs the brain, liver, adipose, pancreas, and skeletal muscle to govern intermediary metabolism. Minute-by-minute adjustments of blood glucose levels involve the combined actions of insulin, glucagon, epinephrine, and cortisol, which activate enzymes to covalently modify key regulatory enzymes. Allosteric modulations also occur in the short term, which presents a feedback measure to regulate enzyme activity.
Long term, the total amount of enzymes can be adjusted to reflect the state of nutrient availability, in either the fed or fasted states. Hormones signal covalent modifications that occur in the cytosol, which differentially induce or suppress proteins that translocate to the nucleus. Here, they up-regulate the transcription of key pathway enzymes.
This integration of metabolism allows the blood glucose level to be maintained through hormonally triggered cascades that counterbalance fluctuations, normally 60 to 90 mg/100 mL, or about 4.5mM due to dietary intake or vigorous exercise.
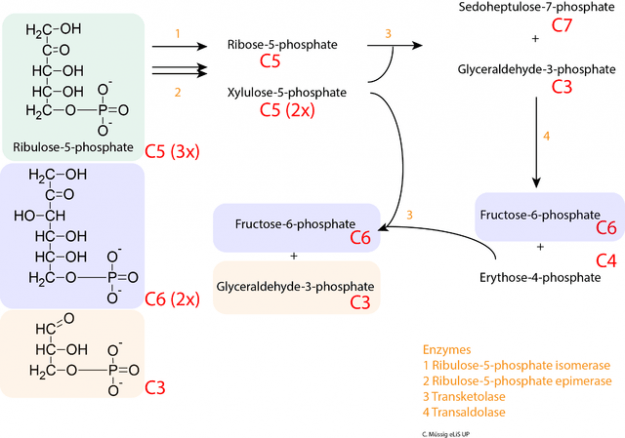
Image: “Stage 2 of the oxidative pentose phosphate pathway (OPPP): conversion of ribulose-5-phosphate into intermediates of glycolysis.” by C. Muessig. License: CC BY-SA 3.0
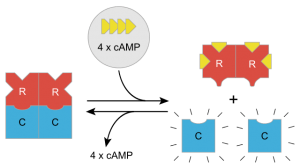
A sketch of activation effects of cAMP on Protein Kinase A
Gene Expression
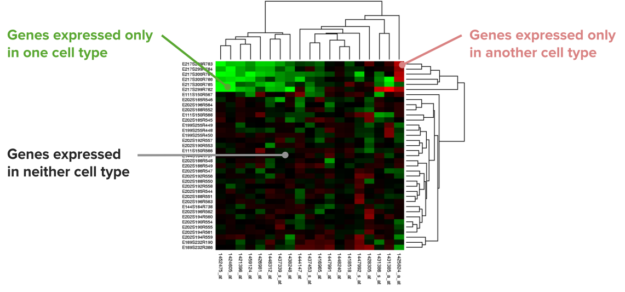